- Docente: Federico Boscherini
- Credits: 6
- SSD: FIS/03
- Language: English
- Moduli: Federico Boscherini (Modulo 1) Francesco Borgatti (Modulo 2) Raffaello Mazzaro (Modulo 3)
- Teaching Mode: Traditional lectures (Modulo 1) Traditional lectures (Modulo 2) Traditional lectures (Modulo 3)
- Campus: Bologna
- Corso: Second cycle degree programme (LM) in Physics (cod. 9245)
Learning outcomes
At the end of the course the student will learn the basic notions regarding the physical mechanisms of the interaction between X-rays and condensed matter in both a macroscopic and microscopic approach and the most important properties of synchrotron radiation sources, with emphasis on the underlying physics. Moreover, the student will learn the basics of the main experimental X-ray methods (such as X-ray diffraction, X-ray absorption spectroscopy and photoemission) and their recent application to current research topics.
Course contents
The objective of the course is to describe the physical mechanisms of the interaction between x-rays and matter and the main experimental methods used in modern research in condensed matter physics and related areas such biophysics, medical physics, cultural heritage and environmental science.
The description of the interaction between x-rays and matter will start with the aid of the damped oscillator model within classical electrodynamics. The interaction will be described both with a macroscopic approach (dielectric function) and an atomic one (atomic form factor); the relation between these approaches will be described, along with the optical theorem, the Kramers - Kronig relations and introductory linear response theory.
X-ray matter interaction will then be described in quantum terms. First of all, the main interaction mechanisms will be described in terms of interaction between photons and atoms: photoelectric absorption (and de-excitation mechanisms), elastic and inelastic scattering. Subsequently, time dependent perturbation theory will be used to derive the cross sections of these processes for hydrogen like atoms. stressing the approximations used and the effects of energy and angular momentum conservation. Finally, the extension to multielectron atoms, molecules and solids will be mentioned.
After a brief description of the constructive elements of electron storage rings, the physical basis underlying the main properties of synchrotron radiations sources will be illustrated; the properties which derive from physical laws, such as the principle of superposition for waves, the relativistic Lorentz transformations and the Heisenber uncertainty principle, and those which are a consequence of the constructive limitations of the accelerators will be distinguished. A brief mention of the new sources based on linear accelerators (free electron lasers) will be made.
The main experimental methods used in current research using synchrotron radiation in condensed matter and applied physics will be described: x-ray diffraction, x-ray absorption and emission spectroscopy and photoemission spectroscopy (this module is the responsibility of Dr. Borgatti).
X-ray diffraction. Classical approach and optical analogy. Elastic x-ray scattering from a free electron and from an atom, the atomic form factor and anomalous corrections. Relation between the scattered intensity and electronic density, the Patterson function and the phase problem. Kinematical theory of diffraction from crystals; Laue conditions, Ewald sphere, Bragg equation, relation to crystal planes, the geometrical structure factor. Experimental methods. Effect of thermal vibrations, the Debye Waller factor. Pair distribution function analysis. Selected examples.
X-ray absorption spectroscopy. Phenomenology. Experimental methods. Main approximations: single electron, dipole, sudden approximation. Wave function of the final state within scattering theory. Scattering amplitude and phase shift. Many body corrections: aplitude reduction factor, mean free path, core hole lifetime. Thermal and structural disorder. Multiple scattering contributions. EXAFS. Fourier transform of EXAFS spectra and its relation to local structure. Examples related to simple crystallin structure. The effect of temperature. XANES: Pre-edge, edge and post-edge regions. Relation between energy position oxidation state (chemical shift); between lineshape and local symmetry. Linear dichroism. The dipole matrix element in the atomic case. Dependence on the relative orientation of polarization and orientation of the final state orbital. Extension to the molecular case, the case of p* orbitals. Selected examples.
Module 1: Photoemission spectroscopy (Dr. F. Borgatti)
(A) Phenomenology of the photoelectric effect. Experimental requirements for photoemission spectroscopy. Energy balance of the process; relaxation and correlation effects; photoemission from a multi-electron system. (B) Cross section of the photoemission process; dipole selection rules; dependence of the cross section on the energy and the polarization of the incident radiation. (C) Overview of Primary and Secondary spectral structures appearing in a spectrum. Core-level photoemission; chemical sensitivity; spin-orbit splitting; lineshape of the peaks. Auger electron emission. Comparison of the main properties of photoelectrons and Auger electrons. Photoemission from valence states; dependence of the spectra on the energy and polarization of incident radiation. (D) Secondary structures in photoemission spectra; multiplet splitting; shake-up and shake-down processes. (E) Photoemission from solid systems; the three-step model. (F) Quantitative analysis; description of contributions to the intensity of the core-level peaks; models for a semi-infinite material and for a thin film. (G) Hard X-ray photoemission; prevalence of the sensitivity to the electronic volume structure over the surface; characteristics of the photoemission cross section for this excitation energy range.
Readings/Bibliography
- Lecture presentations available on iol.unibo.it.
- P. Fornasini, X – ray absorption spectroscopy, available at www.synchrotron-radiation.it (Attività SILS/ scuola di Luce / Grado 2013).
- C. Meneghini, The XANES Region, available at www.synchrotron-radiation.it (Attività SILS/ scuola di Luce / Grado 2013).
Textbooks for in depth description of course topics:
- J. Als – Nielsen and D. McMorrow, Introduction to Modern X-ray Physics, Wiley, New York, 2001.
- D. Attwood, Soft X-rays and extreme ultraviolet radiation, Cambridge University Press (1999).
- A. Balerna and S. Mobilio, Introduction to Synchrotron Radiation, in “Synchrotron Radiation: Basics, Methods and Applications”, a cura di S. Mobilio, F. Boscherini e C. Meneghini, Springer (2015).
- P. Fornasini, Introduction to X-ray absorption spectroscopy, in “Synchrotron Radiation: Basics, Methods and Applications”, a cura di S. Mobilio, F. Boscherini e C. Meneghini, Springer (2015).
- B. Bunker, Introduction to XAFS: a practical guide to X-ray absorption spectroscopy, Cambridge University Press (2010).
- B.E. Warren, X-ray diffraction, Dover, New York, 1990.
- S.J.L. Billinge e E.S. Bozin, Pair distribution function technique: principles and methods, in Diffraction at the nanoscale, a cura di A. Guagliardi & N. Masciocchi, Insubria University Press.
- A. Guinier, X-ray diffraction in crystals, imperfect crystals, and amorphous bodies, Dover, New York, 1994.
- S. Hüfner, Photoelectron Spectroscopy – Principles and Applications, 3rd ed. (Berlin, Springer, 2003)
- C. Mariani e G. Stefani, Photoemission Spectroscopy: fundamental aspectsin “Synchrotron Radiation: Basics, Methods and Applications”, a cura di S. Mobilio, F. Boscherini e C. Meneghini, Springer (2015)
Teaching methods
Lectures with powerpoint presentations, a copy of which is available on the web site iol.unibo.it.
Assessment methods
Oral exam, in two parts. In the first part, each student will illustrate one of the experimental methods (student's choice), focussing on physics fundamentals, experimantal aspects, characteristics and examples. The second part will deal with the fundamental part of the course: properties of x-rays, synchrotron radiation soursces, interaction of x-rays with matter.
The exam must be booked via almaesami, 3 students every hour and a half slot, 12 students a day.
Teaching tools
Presentations. Slides are available to registered students on line
Office hours
See the website of Federico Boscherini
See the website of Francesco Borgatti
See the website of Raffaello Mazzaro
SDGs
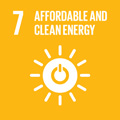
This teaching activity contributes to the achievement of the Sustainable Development Goals of the UN 2030 Agenda.